A Gene Editing Technology Called Crispr-Cas9 Uses Cellular Machinery
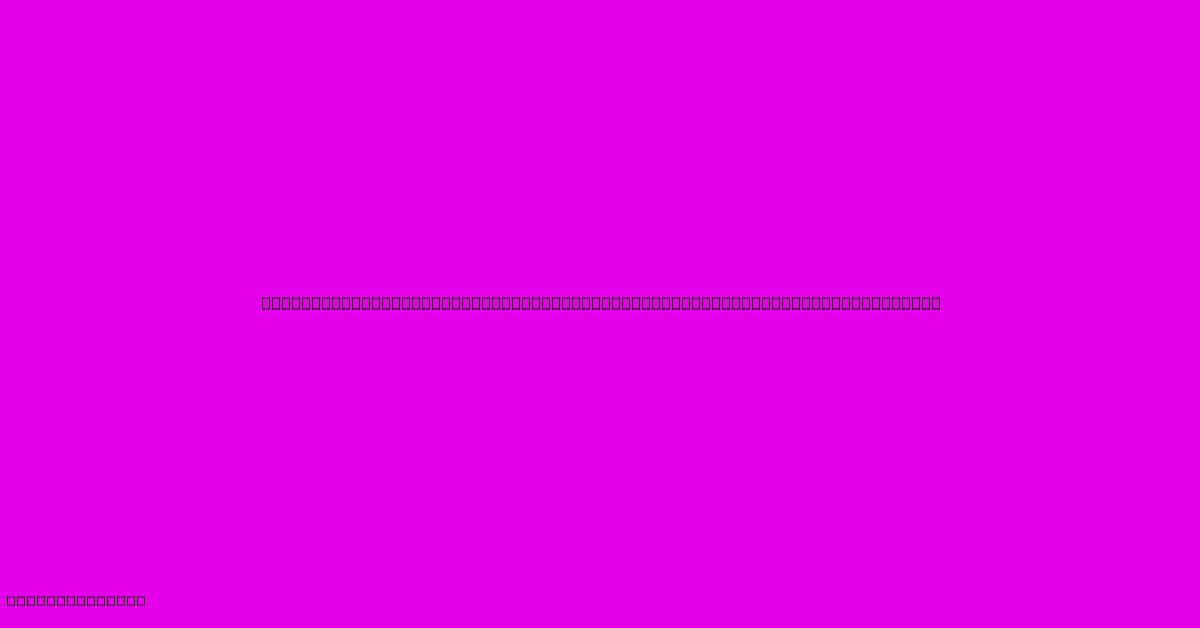
Discover more detailed and exciting information on our website. Click the link below to start your adventure: Visit Best Website mr.cleine.com. Don't miss out!
Table of Contents
CRISPR-Cas9: Revolutionizing Gene Editing with Cellular Machinery
CRISPR-Cas9 has emerged as a groundbreaking gene editing technology, rapidly transforming fields from medicine to agriculture. Its power lies in its ability to precisely target and modify DNA sequences within a cell, leveraging the cell's own machinery to achieve this. This article delves into the mechanisms of CRISPR-Cas9, exploring its applications and potential future impact.
Understanding the CRISPR-Cas9 System
CRISPR-Cas9 is derived from a naturally occurring bacterial defense mechanism against invading viruses. Bacteria utilize CRISPR (Clustered Regularly Interspaced Short Palindromic Repeats) sequences and Cas (CRISPR-associated) proteins to identify and cut viral DNA, effectively disabling it. Scientists have harnessed this system for gene editing by creating an artificial CRISPR-Cas9 complex.
This complex consists of two key components:
- Guide RNA (gRNA): A short RNA molecule engineered to be complementary to a specific target DNA sequence. This acts as a "GPS," guiding the Cas9 protein to the precise location on the genome.
- Cas9 Protein: An enzyme that acts as molecular scissors, cutting both strands of the DNA at the target site specified by the gRNA.
Once the DNA is cut, the cell's natural DNA repair mechanisms kick in. These mechanisms can be exploited in two primary ways:
- Non-homologous end joining (NHEJ): This pathway is error-prone and often results in the insertion or deletion of nucleotides at the cut site, causing a frameshift mutation that disrupts the gene's function. This is useful for gene knockout studies or disabling disease-causing genes.
- Homology-directed repair (HDR): A more precise method that utilizes a provided DNA template to repair the break. This allows scientists to insert specific DNA sequences, correct mutations, or introduce new genes.
Applications of CRISPR-Cas9
The versatility of CRISPR-Cas9 has led to its widespread adoption across numerous scientific disciplines:
1. Medicine:
- Gene therapy: Correcting genetic defects responsible for inherited diseases like cystic fibrosis, sickle cell anemia, and Huntington's disease.
- Cancer treatment: Engineering immune cells (CAR T-cell therapy) to target and destroy cancer cells more effectively.
- Diagnostics: Developing rapid and sensitive diagnostic tools for infectious diseases and genetic disorders.
2. Agriculture:
- Crop improvement: Enhancing crop yields, nutritional value, and resistance to pests and diseases.
- Livestock improvement: Improving animal health, productivity, and disease resistance.
3. Biotechnology:
- Genome engineering: Creating genetically modified organisms (GMOs) with desirable traits.
- Drug discovery: Developing new therapeutic agents and improving existing ones.
Ethical Considerations and Future Directions
While CRISPR-Cas9 holds immense promise, it also raises important ethical concerns, including:
- Off-target effects: The possibility of unintended cuts at locations other than the target site.
- Germline editing: Modifying the genes of reproductive cells, which could have long-term consequences for future generations.
- Accessibility and equity: Ensuring equitable access to CRISPR-Cas9-based therapies.
Despite these challenges, ongoing research focuses on refining the technology to minimize off-target effects, improve targeting accuracy, and develop safer and more efficient delivery methods. Further exploration into the ethical implications and responsible governance of CRISPR-Cas9 is crucial to ensure its beneficial application while mitigating potential risks.
In conclusion, CRISPR-Cas9 represents a transformative advancement in gene editing technology. Its ability to precisely manipulate DNA sequences, utilizing the cell's inherent repair mechanisms, holds vast potential across various fields. As research continues and ethical considerations are addressed, CRISPR-Cas9 is poised to play an increasingly significant role in shaping the future of medicine, agriculture, and biotechnology.
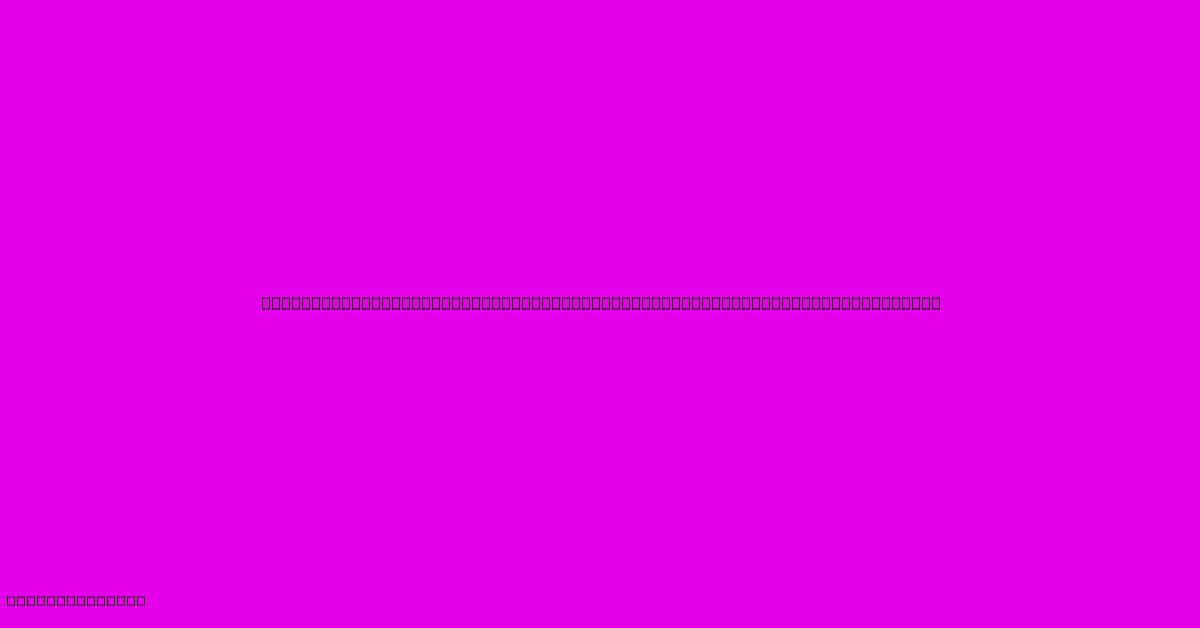
Thank you for visiting our website wich cover about A Gene Editing Technology Called Crispr-Cas9 Uses Cellular Machinery. We hope the information provided has been useful to you. Feel free to contact us if you have any questions or need further assistance. See you next time and dont miss to bookmark.
Featured Posts
-
Fidelity Center For Applied Technology
Jan 01, 2025
-
Joel Klatts 2024 25 Cfp Qf Predictions
Jan 01, 2025
-
Radio Dj Johnnie Walker Dead At 79
Jan 01, 2025
-
Ernst Metal Technologies Llc
Jan 01, 2025
-
My Learning Trane Technologies
Jan 01, 2025